What Symbol Is Used for a Beta Particle? β (Beta)!
In scientific communication, you’ll find beta particles denoted by the symbols β- and β+. These symbols aren’t arbitrary; they provide a precise representation of the particle’s charge and type.
Beta particles, which are high-energy electrons or positrons, play a crucial role in fields like nuclear physics, radiation therapy, and materials science.
Identifying them with β- and β+ ensures clarity, especially when discussing their interactions with matter and their role in processes such as beta decay.
This notation is integral for accurate communication and understanding in science. Grasping the significance of these symbols opens up a deeper insight into nuclear processes.
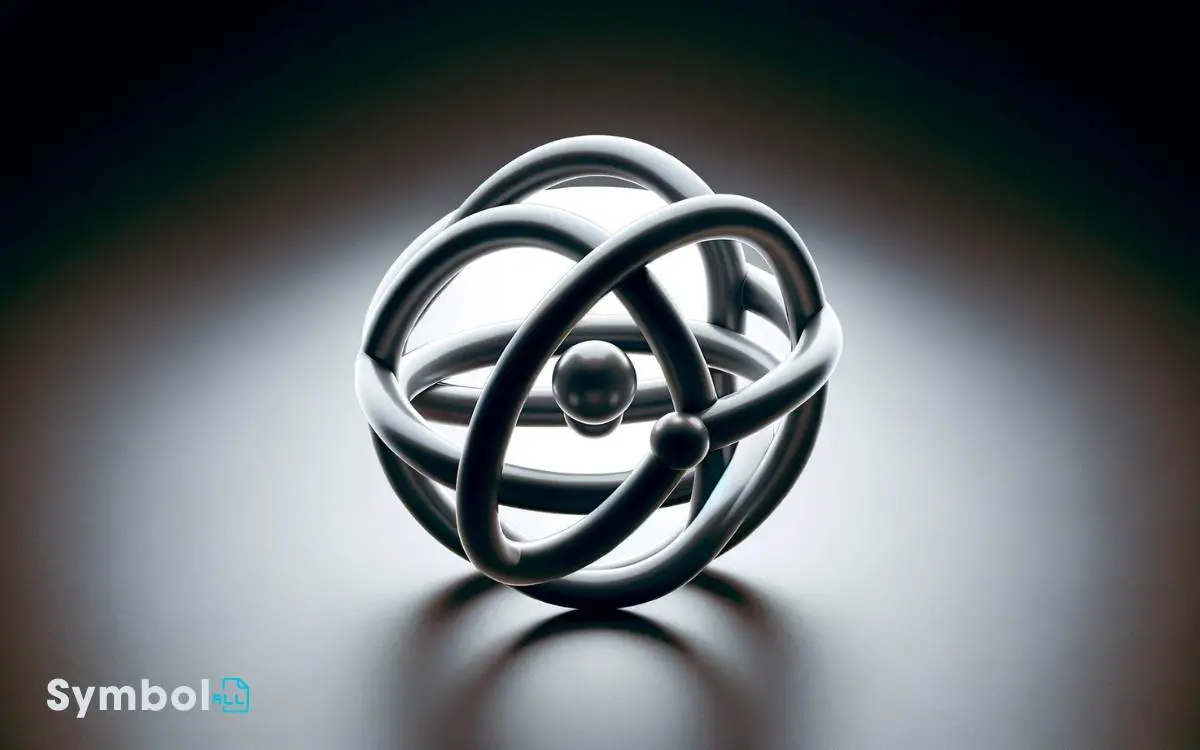
Key Takeaways
Understanding Beta Particles
Beta particles, essentially high-energy, high-speed electrons or positrons, play a pivotal role in nuclear physics and radioactive decay processes.
You’ll find these particles are products of the transformation of neutrons into protons within an atom’s nucleus, a phenomenon critical to understanding the mechanisms behind radioactivity.
They’re characterized by their ability to penetrate living tissue, which is why they’re both a valuable tool and a hazard in medical and industrial applications.
Their interaction with matter, specifically through ionization, is a cornerstone in applications ranging from radiation therapy to material analysis.
As you delve deeper, you’ll appreciate how their behavior underpins crucial principles in both theoretical and applied physics, making your grasp of beta particles foundational to navigating the broader field of nuclear science.
The Symbol Unveiled
Having explored the role and characteristics of beta particles, it’s imperative that you now understand the symbol used to represent them in scientific notation.
Beta particles can be either electrons or positrons emitted during radioactive decay. In scientific literature, the symbols used to denote beta particles are β- and β+ respectively. This distinction is crucial for understanding the type of beta decay a nucleus is undergoing.
Particle Type | Symbol | Charge |
---|---|---|
Electron | β- | Negative |
Positron | β+ | Positive |
These symbols are not just arbitrary; they convey essential information about the particle’s charge, which influences how it interacts with matter and electromagnetic fields.
Mastery of these symbols allows for precise communication and analysis within the scientific community, ensuring clarity in discussions and writings about nuclear physics and radioactivity.
Historical Background
To grasp the evolution of how beta particles have been conceptualized, it’s essential to delve into their historical origins.
Initially identified in the early 20th century, these particles emerged from the study of radioactivity, a field pioneered by researchers like Henri Becquerel and Marie Curie.
Beta particles, or electrons ejected from atomic nuclei during radioactive decay, quickly became a subject of intense investigation. Scientists sought to understand their behavior, energy spectra, and interaction with matter.
This period marked significant strides in nuclear physics, laying the groundwork for the development of quantum mechanics.
Understanding beta particles’ properties and behavior was crucial for unraveling the complex nature of atomic and subatomic processes, thus propelling forward the broader scientific understanding of the physical world.
Symbol Significance
Building on the historical understanding of beta particles, it’s important to examine the significance of their symbol in scientific notation and communication.
The symbol for a beta particle, β, isn’t just an arbitrary choice; it conveys specific information about the particle’s nature and behavior in a concise manner.
In scientific discourse, symbols serve as a universal language, enabling researchers from diverse backgrounds to share and interpret data with precision.
The β symbol denotes electron emission in nuclear processes, distinguishing it from other types of radiation. This distinction is crucial for understanding the mechanisms of radioactive decay and the properties of the emitting nucleus.
By adopting the β symbol, scientists ensure clarity and avoid misunderstandings, facilitating accurate communication and further research in the field.
Beta Decay Explained
Beta decay represents a fundamental nuclear process where an unstable atom transforms by emitting a beta particle, either an electron or a positron, to reach a more stable state.
This transition is crucial for understanding atomic behavior and the forces at play within the nucleus.
- Conservation of Charge: The charge is conserved, with the emitted particle balancing the nuclear charge change.
- Neutrino Emission: Accompanies beta particle emission, carrying away excess energy and momentum.
- Nuclear Forces: Highlight the interplay of weak nuclear forces in beta decay processes.
- Energy Release: Characterized by the release of kinetic energy, shared between the emitted beta particle and neutrino.
- Transformation: Results in the conversion of a neutron to a proton or vice versa, altering the atomic number.
Variants of Beta Particles
In understanding the nuances of beta decay, it’s essential to recognize two primary variants of beta particles: the beta minus (β-) and the beta plus (β+), each playing a distinct role in nuclear transformations.
β- decay involves a neutron transforming into a proton, emitting an electron (β-) and an antineutrino. Conversely, β+ decay occurs when a proton converts into a neutron, releasing a positron (β+) and a neutrino.
This distinction is critical as it highlights the balance process within atomic nuclei, ensuring stability through the conversion of protons to neutrons or vice versa.
Understanding these variants allows you to grasp the fundamental mechanisms that govern radioactive decay, furthering your comprehension of both natural and artificial nuclear processes.
Symbol Usage in Physics
Physics’ use of symbols, including those for beta particles, serves as a concise language for conveying complex concepts and processes efficiently. This symbolic approach allows you to grasp the fundamentals and intricacies of physics with greater ease.
It’s vital to understand the purpose behind this symbolism:
- Symbols provide a universal language that transcends linguistic barriers.
- They enable the succinct expression of mathematical relationships and physical laws.
- Symbols facilitate the visualization of concepts that are abstract or not directly observable.
- The use of symbols enhances precision in scientific communication, reducing ambiguity.
- They allow for the compact representation of complex equations and theories, making analysis and problem-solving more manageable.
Grasping these aspects, you’ll appreciate the depth and complexity symbols, like that for beta particles, add to the field of physics.
Mathematical Representation
As you explore the mathematical representation of beta particles, it’s crucial to understand the notation and symbolic interpretation used within this context.
The symbols employed serve as a concise language, communicating complex physical phenomena efficiently.
Grasping this notation is key to deciphering the underlying principles of beta decay and particle interactions.
Beta Particle Notation
Understanding the notation for a beta particle involves recognizing its representation as either a high-energy, high-speed electron (\( eta^- \)) or positron (\( eta^+ \)) in nuclear equations.
- \( eta^- \): Represents a beta particle that’s an electron. It signifies a negative charge.
- \( eta^+ \): Denotes a beta particle that’s a positron, indicating a positive charge.
- Mass: Both types have a negligible rest mass compared to protons or neutrons, yet it’s crucial for energy calculations.
- Charge: The charge is essential in determining the particle’s interaction with magnetic and electric fields.
- Energy: Beta particles exhibit a wide range of energies, influencing their penetration depth in materials.
Your grasp of these symbols allows for precise communication and understanding in the realm of nuclear physics.
Symbolic Interpretation
Delving into the mathematical representation, you’ll find that the symbolic interpretation of beta particles is crucial for accurate calculations in nuclear physics.
Beta particles, represented by the symbols \(eta^-\) for negatively charged electrons and \(eta^+\) for positrons, play a significant role in understanding nuclear reactions and decay processes.
The negative or positive superscript denotes the charge of the particle, a fundamental aspect that influences how these particles interact with matter and electromagnetic fields.
In calculations, these symbols allow physicists to concisely express and predict the outcomes of beta decay processes. For instance, when a neutron transforms into a proton, a \(eta^-\) particle is emitted.
This notation isn’t merely symbolic but underpins the quantitative analysis of beta decay, enabling precise predictions of energy release and particle trajectories.
Comparing Beta to Other Particles
You’ll find that beta particles, unlike alpha particles, carry a single electronic charge and have a significantly lower mass, highlighting a fundamental disparity in their physical properties.
When comparing beta particles to gamma radiation, it’s crucial to note that gamma rays are massless energy packets, contrasting sharply with the material nature of beta particles.
This distinction is pivotal in understanding their interactions with matter, as charge and mass heavily influence penetration depth and ionization potential.
Beta Vs Alpha Particles
Beta particles differ from alpha particles in several key characteristics, including mass, charge, and penetration capabilities. They’re less massive, carry either a positive or negative charge, and can penetrate materials more deeply than their alpha counterparts.
Here’s a closer inspection:
- Mass: Beta particles are electrons or positrons with significantly less mass than the helium-4 nuclei composing alpha particles.
- Charge: Alpha particles carry a positive charge (+2), while beta particles can be negatively charged (electron) or positively charged (positron).
- Penetration capabilities: Beta radiation can penetrate further into materials than alpha radiation, requiring more substantial shielding.
- Interaction with matter: Beta particles interact through ionization and excitation, leading to different safety considerations.
- Source: They originate from the nucleus during radioactive decay, similar to alpha particles, but involve different processes.
Gamma Radiation Differences
Unlike beta particles, gamma rays possess no mass or charge, enabling them to penetrate materials more deeply and posing unique shielding challenges. You’re now navigating the realm of radiation types, where understanding the nuances is crucial.
Gamma rays, unlike their particle counterparts, require dense materials like lead for effective shielding. This is due to their high penetration power, directly linked to their lack of mass and charge.
Property | Beta Particles | Gamma Rays |
---|---|---|
Mass | Yes | No |
Charge | Yes | No |
Penetration | Moderate | High |
Shielding | Lighter materials | Dense materials |
Interaction | With matter | With matter and electromagnetic fields |
This table encapsulates the fundamental differences, guiding you through the complexities of radiation types with precision.
Charge and Mass Comparison
- Beta particles have a charge of -1 (for electrons) or +1 (for positrons), distinguishing them sharply from neutral gamma rays and neutrons.
- In terms of mass, beta particles are significantly lighter than alpha particles, with electrons and positrons having a mass approximately 1/1836 that of a proton.
- Unlike gamma rays, which are massless energy packets, beta particles possess a tangible mass, contributing to their distinct interactions with matter.
- Alpha particles, being helium nuclei, are substantially more massive and carry a +2 charge, making their behavior in magnetic and electric fields contrast markedly with that of beta particles.
- Neutrons, carrying no charge, interact differently with materials compared to the charged beta particles, leading to variations in penetration and absorption properties.
This comparison highlights the unique attributes of beta particles in the diverse world of radiation particles.
Practical Applications
Radiation therapy’s utilization of beta particles plays a pivotal role in modern cancer treatment strategies, offering targeted and effective tumor destruction.
These subatomic particles, emitted by certain radioactive materials, have the capability to penetrate living tissues to a limited extent, making them ideal for treating superficial tumors without causing extensive damage to the surrounding healthy tissues.
Your understanding of this process hinges on recognizing the balance between the beta particles’ energy and their penetration depth. Too little energy, and they can’t reach the tumor; too much, and they may harm more than just the target cells.
This precision allows oncologists to craft treatments that minimize side effects while maximizing the therapeutic outcome, demonstrating the critical role of beta particles in advancing cancer therapy.
Further Reading and Resources
To deepen your understanding of beta particles and their applications in radiation therapy, it’s essential to consult a variety of scientific publications and online resources.
These materials will provide you with a comprehensive overview, from the basic physics of beta radiation to its practical applications in medical treatments.
To guide you in your research, here are five key resources:
- Principles of Nuclear Medicine by H. Lee and Z. H. Cho
- Radiation Oncology: A Physicist’s-Eye View by Michael Goitein
- Introduction to Radiological Physics and Radiation Dosimetry* by Frank Herbert Attix
- The International Atomic Energy Agency (IAEA) website for guidelines on radiation safety and therapy
- PubMed and Google Scholar for peer-reviewed articles on the latest research in beta particle therapy
Each of these resources offers unique insights, ensuring you gain a thorough understanding.
Conclusion
You’ve navigated the intricate world of beta particles, unearthing the symbol β as their flag. This journey, akin to splitting the atom of knowledge, has showcased β’s role in the grand tapestry of physics.
Through the lens of beta decay, you’ve compared its characteristics against its particle brethren, uncovering its unique applications. Armed with this precise, analytical insight, you’re now better equipped to dive deeper into the atomic realm, where β plays a pivotal part.